Introduction
Enzymes are specialized proteins acting as nature’s catalysts, facilitating biochemical reactions that would otherwise proceed far too slowly to sustain life. From digestion to DNA replication, enzymes play a crucial role in nearly every biological process. Their importance has transcended biology, as industries worldwide increasingly rely on enzymes to enhance production in pharmaceuticals, biofuels, and food processing.
However, industrial use often demands that enzymes operate in extreme conditions, which presents unique challenges. This essay explores enzyme types, their chemistry, the industrial conditions they face, and the hurdles in maintaining enzyme production in favorable conditions, as well as the packaging innovations that allow for their practical use.
Types of Enzymes and Their Chemistry
Enzymes are highly diverse, categorized primarily based on the reactions they catalyze. Here are some of the main types:
Hydrolases
Function: Hydrolases catalyze reactions involving water, breaking bonds in molecules by adding water (hydrolysis). They play a role in digestion, breaking down complex molecules like proteins and carbohydrates into simpler forms.
Example: Proteases, which break down proteins, are essential in the food and detergent industries for their role in protein degradation.
Oxidoreductases
Function: These enzymes facilitate oxidation-reduction (redox) reactions, where electrons are transferred from one molecule to another.
Example: Catalases, which help neutralize hydrogen peroxide in cells, are used in textiles to remove excess peroxide after bleaching.
Transferases
Function: Transferases catalyze the transfer of functional groups (like a phosphate or methyl group) from one molecule to another.
Example: Kinases, critical in energy transfer within cells, are useful in pharmaceutical production for synthesizing drugs that require specific chemical modifications.
Isomerases
Function: These enzymes rearrange the structure of molecules to form isomers, which have the same chemical formula but a different structure.
Example: Glucose isomerase, widely used in food processing, converts glucose into fructose, a sweeter sugar variant, used in high-fructose corn syrup.
Lyases
Function: Lyases break bonds by mechanisms other than hydrolysis and oxidation, often forming double bonds or rings in the process.
Example: Decarboxylases, which remove carboxyl groups, are useful in various biochemical and industrial processes, such as fermentation.
Ligases
Function: Ligases join two molecules together, often requiring an energy source like ATP.
Example: DNA ligase, vital in biotechnology and genetic engineering, allows researchers to insert genes into DNA for cloning or other genetic modifications.
Each enzyme’s chemistry involves an intricate structure, where the “active site” binds specific substrates to catalyze reactions. This specificity makes enzymes incredibly efficient but also sensitive to environmental conditions.
Extreme Industrial Conditions and Enzyme Durability
Industrial applications often expose enzymes to conditions they wouldn’t naturally encounter, such as:
High Temperatures: Many processes require temperatures exceeding 70°C to increase reaction rates. However, enzymes denature, or lose their structure, at high temperatures, making them inactive.
Variable pH Levels: Industrial reactions may occur in acidic or basic environments that are too harsh for most enzymes, which typically function best at neutral pH.
High Pressure: Some reactions require elevated pressures to accelerate reactions, which can destabilize enzyme structures.
Organic Solvents: Many industrial processes involve organic solvents that dissolve fats or oils, but they often disrupt the delicate structure of enzymes, rendering them inactive.
Certain enzymes, such as those from thermophilic (heat-loving) organisms, have evolved to withstand extreme conditions, making them better suited for high-temperature processes. However, these natural “extremophiles” are limited, and industrial-scale production of such enzymes remains challenging.
Challenges in Producing Enzymes in Optimal Conditions
It would seem ideal to produce enzymes in the conditions where they thrive. However, replicating these natural conditions on an industrial scale poses significant challenges:
Cost and Resources: Creating and maintaining environments similar to those of extremophiles is costly. Industrial facilities would need special equipment and materials to sustain high temperatures, unique pH levels, or other conditions, which would increase production costs significantly.
Yield and Efficiency: Even if enzymes are produced in favorable conditions, yields can be lower due to the specific needs of extremophiles, which might require longer growth times or specialized nutrient profiles.
Genetic and Environmental Constraints: Genetic engineering has allowed for the modification of enzymes to make them more resilient, but there are limits. Many enzymes, despite being modified, still perform best in mild, non-extreme conditions, limiting their usability in high-stress industrial processes.
Scalability: Maintaining controlled environments for enzyme production is complex, especially when scaling up for mass production. For instance, fermentation tanks need precise temperature and pH regulation, which becomes exponentially challenging in large volumes.
These challenges mean that while some enzymes are engineered for durability, producing enzymes in entirely favorable conditions is rarely feasible at an industrial scale.
Enzyme Packaging and Stabilization for Industrial Application
To overcome these obstacles, industries have developed innovative methods for enzyme packaging and stabilization, allowing enzymes to withstand harsher environments:
Encapsulation: Encapsulation involves surrounding enzymes with protective materials that shield them from extreme conditions. This can be done with polymers, lipids, or other materials that protect enzymes from temperature, pH changes, or solvents. Encapsulated enzymes are particularly popular in laundry detergents, where they remain active in a range of temperatures.
Cross-Linking: Cross-linked enzyme aggregates (CLEAs) involve binding enzymes together with cross-linkers to form stable clusters. These clusters are more resistant to denaturation and can be used in processes with higher temperatures and pressures.
Immobilization: Immobilization attaches enzymes to solid supports, which helps maintain their structure and activity over repeated uses. This method is widely used in pharmaceuticals, where immobilized enzymes can be used for multiple reaction cycles, reducing costs.
Lyophilization (Freeze-Drying): Freeze-drying enzymes helps preserve them in a stable, powdered form. This is especially useful for storage and transportation, as lyophilized enzymes have a longer shelf life and can be rehydrated when needed.
These methods allow enzymes to function effectively in conditions that would otherwise degrade them, making it possible for industries to harness their catalytic power despite environmental challenges.
Conclusion
Enzymes are invaluable assets to biotechnology, enabling efficient, specific, and often environmentally friendly reactions. Their chemistry allows them to catalyze reactions with extraordinary precision, yet industrial demands expose them to extreme conditions that push the limits of their resilience. While producing enzymes in naturally favorable conditions is a logical goal, practical challenges make it difficult to implement on a large scale.
The advancements in enzyme packaging and stabilization, however, offer promising solutions that allow enzymes to withstand harsh environments and extend their industrial utility.
As biotechnology advances, so too will our ability to engineer and apply enzymes in diverse settings, bridging the gap between nature’s delicate design and the rigorous demands of industry.
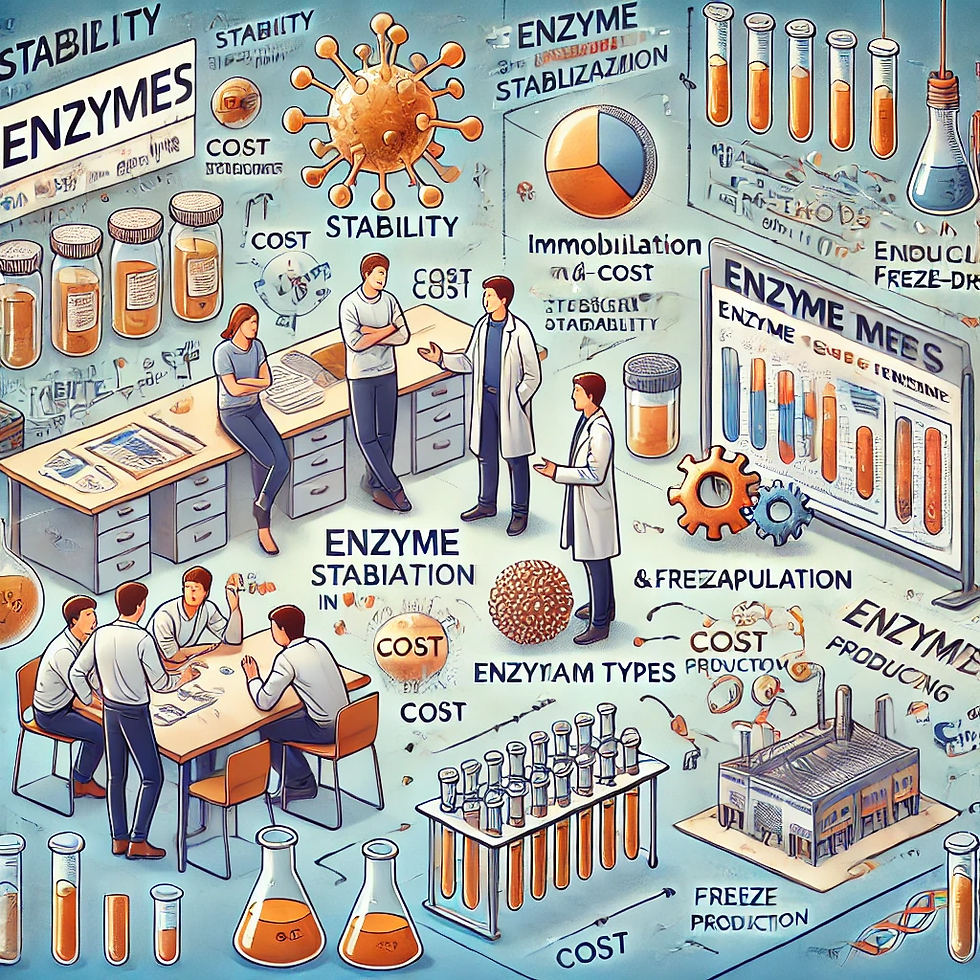
Brainstorming Session: Young Researchers Discussing Enzyme Challenges in Industry
Participants: Emily (Biotech Engineer), Raj (Chemistry PhD Student), Sara (Biochemist), Leo (Industrial Engineer), Mei (Molecular Biologist)
Emily: Thanks for joining, everyone! So, our topic today is the use of enzymes in industrial processes. I thought we could start by discussing what we each see as the biggest challenge with industrial enzyme use.
Raj: I’d say it’s stability. Enzymes are fantastic in lab settings, but they often don’t survive the extreme conditions in industrial applications. High temperatures, extreme pH, and solvents can denature them pretty quickly.
Sara: Definitely! And the irony is, enzymes are so precise and efficient—until they’re exposed to those conditions. It’s almost like we’re putting them in their worst-case scenarios to get the benefits. But what if we could modify enzymes to make them tougher?
Leo: That would be amazing, but modifying enzymes can also mess with their activity. I’ve seen cases where engineering enzymes for stability actually reduced their catalytic efficiency. It’s a tricky balance.
Mei: True. Another option we’ve been exploring is using extremophiles—organisms that already survive in these harsh environments. Their enzymes are naturally stable under extreme conditions, but isolating and mass-producing those enzymes is a whole different challenge.
Emily: Right, and then there’s the cost. Industrial-scale production of extremophile enzymes could be expensive, especially if you’re trying to keep everything controlled to replicate their native environments.
Raj: It’s like we’re caught between optimizing conditions for enzymes or optimizing enzymes for conditions. One idea I read about recently was enzyme immobilization, where enzymes are attached to solid supports. It can help protect them and even allow for reuse, which is great for cost efficiency.
Sara: I’ve used immobilization techniques in a few experiments. It does help stabilize the enzyme, but it’s not a one-size-fits-all solution. Some enzymes don’t bind well to supports, or their activity is reduced when they’re immobilized.
Mei: Speaking of stabilization, what about encapsulation? We could encapsulate enzymes in materials that shield them from extreme conditions. Polymers or even lipid-based capsules can protect them from pH changes or high temperatures.
Leo: That could work! I could see encapsulation being really useful for enzymes used in detergents, where they need to withstand both hot and cold water temperatures. But encapsulation adds another step and can be pricey.
Emily: So maybe there’s a hybrid solution? We could combine different stabilization methods based on the specific requirements of the industrial process. For example, immobilizing enzymes for repeated reactions in pharmaceutical production, and encapsulating them for detergents or food processing.
Raj: And on the cost side, maybe there’s room for more automation or scaling innovations in enzyme production? If we can lower production costs, it might make it easier to justify the expense of stabilization techniques.
Sara: That’s a good point. Another idea might be producing enzymes in bulk using recombinant DNA technology. This could give us more control over enzyme characteristics while reducing costs. But that still leaves the problem of how to maintain activity under extreme conditions.
Mei: What if we designed synthetic enzymes that mimic the natural ones but with added stability? I know it’s a bit futuristic, but advances in protein design might let us create enzyme-like molecules that are more robust.
Leo: Synthetic enzymes are a fantastic idea! Though they’re complex to develop, they could potentially handle conditions that natural enzymes can’t. This could be revolutionary for industries where extreme conditions are non-negotiable.
Emily: Yes, and if we combine synthetic enzymes with natural extremophile ones, we could build a toolkit for different industrial uses. Plus, it gives us a broader set of options depending on the specific challenges in each industry.
Raj: Good thinking! So, just to summarize, we’re talking about several possible solutions:
Genetic modification of enzymes for stability.
Using enzymes from extremophiles.
Immobilization and encapsulation for protection.
Recombinant DNA to reduce costs.
Developing synthetic enzymes as a long-term solution.
Sara: That sounds like a solid framework. But what about storage and transport? If we’re dealing with enzymes that are sensitive to environmental changes, we’ll need to think about packaging.
Mei: Maybe freeze-drying or lyophilization? Freeze-dried enzymes have a longer shelf life and are easier to transport. They can be rehydrated right before use, so they’re fresh and active.
Leo: Lyophilization could work, especially for enzyme kits sent to remote facilities or international locations. It could simplify transport and storage logistics.
Emily: I like it! So, let’s keep exploring the best applications for each method. It looks like we’ve got a great starting point for some practical solutions. Thanks, everyone! This was super insightful.
Summary of Ideas:
Stabilization Techniques:
Genetic Modification for heat and pH tolerance.
Immobilization for reuse and stability in pharmaceutical applications.
Encapsulation for temperature and solvent protection in detergents and food processing.
Enzyme Production:
Extremophiles for natural stability under extreme conditions.
Recombinant DNA to reduce costs and modify enzymes for industrial needs.
Synthetic Enzymes as a future solution for extreme environments.
Packaging and Transport:
Freeze-Drying for long shelf life and easy rehydration.
Lyophilization for simplified storage and transport.
Comments